This research was published in the INSPIRED: A Publication of the New Hampshire Agricultural Experiment Station (Fall 2024)
Researchers: M. Glenn, A. Mathieson, R. E. Grizzle & D. Burdick
Oyster farming has rapidly grown in New Hampshire's Great Bay Estuary. In addition to strengthening the regional food system, shellfish aquaculture can provide other ecological benefits by increasing seaweed biodiversity and habitat services to the broader ecosystem. However, there's been uncertainty about the extent to which gear for farmed oyster operations may aid or detract from seaweed biodiversity, especially when compared to three natural subtidal habitats—an oyster reef, eelgrass bed, and mudflat.
Background and Key Concepts
Oyster aquaculture has seen rapid growth in the Great Bay Estuary (GBE), with production increasing significantly over the past decade. In 2013, New Hampshire oyster farmers harvested 81,274 oysters, which grew to 821,157 oysters by 2022, reflecting a more than 1,000 percent increase in the industry's value since 2013. This expansion necessitates a deeper understanding of the ecological impacts of oyster farming, particularly the role of farm gear in altering marine habitats.
Previous studies have shown that oyster farm gear increases habitat complexity, supporting diverse marine communities and promoting biodiversity, which is essential for the sustainable development of the industry.
Seaweed biodiversity is crucial for the health of estuarine ecosystems. Seaweeds provide food and habitat for numerous marine organisms, contribute to nutrient cycling, and help stabilize sediments. Diverse seaweed communities support higher productivity and resilience, offering ecosystem services such as water purification, coastal protection, and enhanced habitat for fish and invertebrates. Understanding the interactions between seaweed and oyster farm gear can inform better management practices and enhance the ecological benefits of aquaculture.
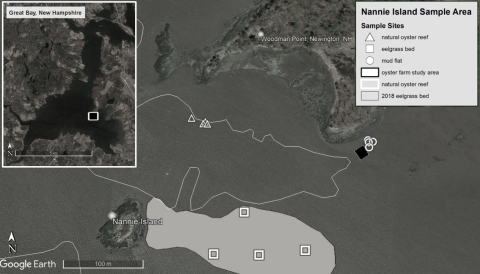
Fig. 1. Study area in Great Bay, New Hampshire and locations of sample sites on natural oyster reef and in eelgrass bed.
Key Findings
- In New Hampshire's Great Bay, oyster farm gear and eelgrass beds supported the greatest number of seaweed species.
- Seaweed biomass was also greater on oyster farm gear than in natural habitats, particularly mudflats, demonstrating the gear's capacity to support dense seaweed growth.
- Non-native seaweeds dominate the biomass on oyster farm gear and mudflats, comprising over 80% of the total biomass.
About the Co-author
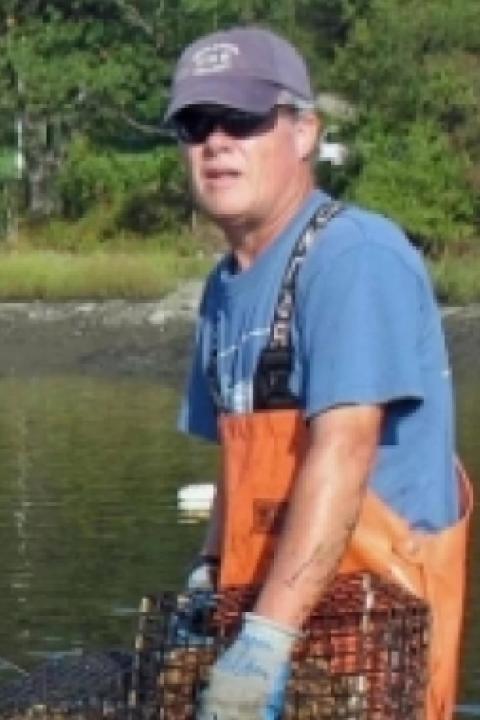
Raymond Grizzle, Research Professor of Biological Sciences
Contact information: Raymond.Grizzle@unh.edu, FindScholars profile
Methodology

Fig. 2. Oyster rack ("farm gear") used to hold oyster bags. Racks in this photo are empty, bags would be located on each of the three levels. Sampling device used to extract samples from all four study habitats.
The study was conducted in the Great Bay Estuary, a mesohaline, macrotidal system in New Hampshire with a tidal range of approximately 2.7 meters. Four subtidal habitats (Fig. 1) were examined: oyster farm gear, an oyster reef, an eelgrass bed, and a mudflat. To simulate the farm gear habitat, 12 replicate cubical oyster racks were deployed (Fig. 2) on a mudflat, each holding three bags containing approximately 190 oysters. The natural habitats were sampled using a custom-made device capturing a standard area of 0.25 m².
Sampling occurred in August and October 2014, and in August 2015. Four replicates were taken per habitat on each sampling occasion, totaling 12 replicates per habitat. Collected seaweeds were sorted, identified, and weighed in the laboratory. Statistical analyses, including ANOVAs and multivariate analyses, were conducted to compare species richness, biomass, and community composition among the habitats.
Discussion of Findings
The study revealed significant differences in seaweed species richness and biomass across the four habitats. Eelgrass beds supported the highest species richness, with an average of 35 species, followed by oyster reefs with 28 species and oyster farm gear with 25 species. Mudflats exhibited the lowest species richness, averaging only 12 species (Fig. 3). This indicates that the structural complexity of oyster farm gear provides a favorable environment for diverse seaweed communities.

Fig. 3A.Biomass distribution across habitat types, represented as dry weight (g) per 0.25 m² plot. The graph differentiates between red algae (hashed bars) and green algae (solid bars), with mean values and standard error included. Data from all sampling dates were averaged (N = 12), showing significant habitat-based variations.
Biomass comparisons showed a similar trend. Oyster farm gear had the highest biomass, significantly greater than that of eelgrass beds, oyster reefs, and mudflats. This suggests that the vertical structure and hard surfaces provided by farm gear enhance seaweed growth, supporting dense algal communities. Mudflats had the lowest biomass, reflecting their less complex habitat structure.
The analysis of native versus non-native species distribution revealed that non-native seaweeds dominated the biomass on oyster farm gear and mudflats, comprising over 80% of the total biomass in these habitats. Conversely, native species were more prevalent in eelgrass beds and oyster reefs. Notably, the non-native species Agarophyton vermiculophyllum, Gracilaria tikvahiae, and Ulva lactuca contributed to the high biomass on farm gear and mudflats.
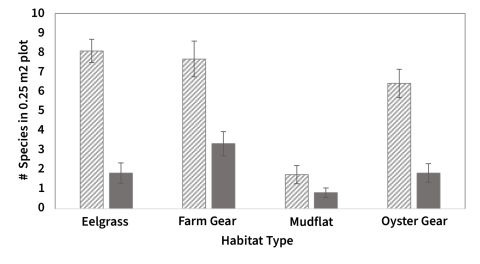
Fig. 3B. Comparison of species richness across different habitat types, with red algae (hashed bars) and green algae (solid bars) shown separately. The mean number of species per 0.25 m² plot is presented with standard error, averaged over all sampling dates (N = 12). Significant differences were observed among habitats.
Strategic Recommendations and Conclusion
The study underscores the ecological benefits of oyster farm gear in supporting diverse seaweed communities, indicating its potential for enhancing biodiversity in the GBE.
Recommendations for Enhancing Biodiversity through Aquaculture Practices:
- Farm Gear Design: Use gear that maximizes habitat complexity to support diverse seaweed communities, including vertical structures and hard surfaces.
- Monitoring Non-Native Species: Regularly monitor seaweed communities to manage non-native species effectively and mitigate potential negative impacts.
- Integration with Restoration Projects: Integrate oyster farming with eelgrass and oyster reef restoration efforts to enhance overall ecosystem health.
Policy Considerations for Sustainable Oyster Farming:
- Comprehensive Management Plans: Develop plans recognizing oyster farms' dual role in aquaculture and biodiversity enhancement.
- Stakeholder Collaboration: Foster collaboration between farmers, researchers, and regulatory agencies to implement best practices and address challenges.
By integrating these recommendations, oyster farming can contribute to regional biodiversity and ecosystem services while supporting the aquaculture industry's growth.
This material is based on work supported by the NH Agricultural Experiment Station through joint funding from the USDA National Institute of Food and Agriculture (under Hatch award number 1013469) and the state of New Hampshire.